Antarctica and the Wandering Poles
- Leenie Wilcox
- Dec 14, 2024
- 6 min read
Updated: Jan 29
** Note that this is a lecture originally written for my high school physics students **
Ferromagnetism
If you've ever played with a refrigerator magnet or a bar magnet of some kind, then you know that a magnet has the ability to exert a force. By holding the magnet above a pile of safety pins, you might be able to pick up a few, or create a chain. Perhaps you've also tried forcing two magnets together, and can recognize that the `north' or `south' poles repel each other, while a `north', `south' combination immediately wants to attach.
Permanent magnets like this are called ferromagnets (or, a slightly different permanent magnet, ferrimagnets). These kinds of magnets work by having each molecule's magnetic dipole align. The combination of all these microscopic magnetic dipoles makes the overall magnet we can feel the strong force of. This is why iron, for example, creates a magnetic field regardless of the external circumstances.

The magnetic field of a permanent magnet (bar magnet) looks something like figure 1.
These lines, similar to the electric field lines we have seen earlier, show the direction and strength of the magnetic field. If I were to place a compass beside a bar magnet, the needle of the compass would align with the magnetic field, and point along the field lines. This is how we use compasses to navigate on Earth; by a compass needle aligning with the Earth's magnetic field.
These lines, similar to the electric field lines we have seen earlier, show the direction and strength of the magnetic field. If I were to place a compass beside a bar magnet, the needle of the compass would align with the magnetic field, and point along the field lines. This is how we use compasses to navigate on Earth; by a compass needle aligning with the Earth's magnetic field.
Moving Charge
However, while there is a lot of iron in the Earth, ferromagnetism isn’t how the Earth creates its magnetic field.
Another way to create a magnetic field is by moving electrical charges [4]. The strength of the magnetic field depends on how much charge is moving, and how quickly that charge is moving. This is called current, and is quite like what you might think of when talking about rivers or channels of water.
The magnetic field produced by a current is given by the Biot-Savart law, and for a line of current, it looks like this:

Now, we haven’t touched integrals, but if you look at this equation, I want you to see:
Magnetic Field as you move radially away = constants multiplied by a calculus thing which includes a cross product of the current with some distance away from the current.

We’ve become used to the idea that the cross product is a way of multiplying vectors, but this is perhaps the first time we have seen it out in the wild, not as a plain math practice problem. A useful tool to visualize how the cross product
works is the Right Hand Rule. In the right hand rule, you use your right thumb, pointer finger, and middle finger. Point your thumb in the direction of the first vector, your pointer finger in the direction of the second, and make a right angle from your middle finger to your pointer finger. The direction your middle finger is pointing is the direction of your new vector!
As an example, if I create a current going down a straight wire, and define the r direction to be going radially away from the wire, then I can use the Right Hand Rule (RHR) to figure out that the magnetic field is going in circles centered around the wire!
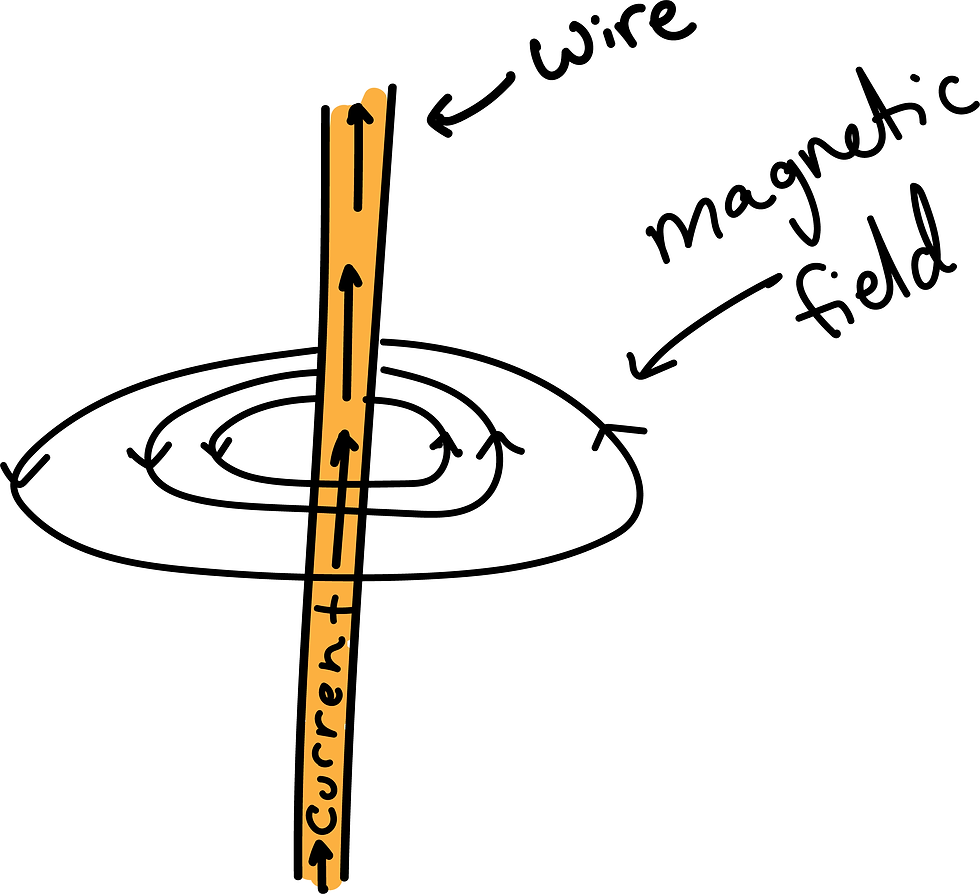
In The Earth’s Core
The Earth is like an ogre. It has layers.
The top layer is the crust, which is what we walk on and what childhood Leenie would try to dig through to get to China [5]. The next layer down is the mantle, which is hot, pressurized, solid rock (though it is often described as caramel-y, or plastic since on long enough time scales it behaves like a liquid). Then comes the core, which is made of iron. The outer core is a liquid, and the inner core is under so much pressure that it is solid iron.
The core radiates heat through two processes; conduction and convection. Conduction is the direct transfer of heat through physical contact. This is like putting your hand on a cold lamppost. Convection is heat transfer through the movement of a fluid. This is like when water boils. The water at the bottom of the pot is closer to the stove top, and gets hot faster than the water at the top of the pot. This hotter water becomes less dense by expanding, and the cold water at the top (which is more dense) sinks to the bottom of the pot. This creates a sort of loop, where the water moves in vertical circles through the pot, transferring heat from the bottom of the pot to the top. This is what happens in the Earth’s outer core and mantle.
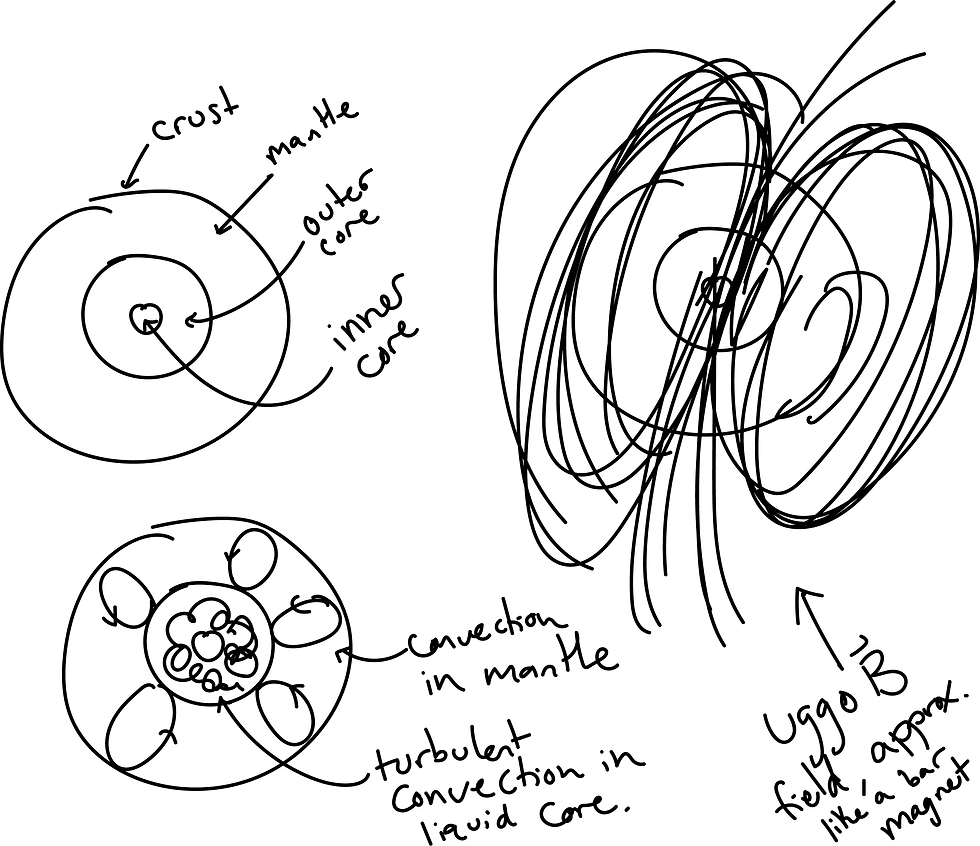
The liquid portion of the core is extremely turbulent, and generates electrical charge through radioactivity and chemical differentials [7, 3]. If these charges move, they turn into electrical currents.
The core creates a magnetic field which is continually responding to its own internal chaotic feedback [3]. The newly generated electrical charge reacts to the existing magnetic field, and when this charge moves it creates its own current and its own magnetic field. The process, therefore, is cyclic, and the magnetic field is able to be maintained as long as the core generates enough energy [3].
Although the Earth’s magnetic field does look like a bar magnet on the whole, you may imagine that unlike a static bar magnet, this turbulent liquid core might create a magnetic field which is not perfectly static.
And you’d be right.
The Quest For The Pole
In 1907, Ernest Shackleton set out on an expedition to Antarctica on the Nimrod [1][6, p 129]. It was an expedition with multiple objectives, one of which was to reach and plant a flag at the magnetic south pole. The magnetic south pole had been attempted several times, along with the geologic south pole. The geologic south pole is aligned with the Earth’s axis of rotation, and it was known at the time that the magnetic and geologic poles didn’t perfectly align.
Shackleton assigned three men, David Edgeworth, Dr. Alistair Mackay, and Douglas Mawson to set out for the magnetic pole [6, p 191]. The men had to traverse a total of 1260 miles [1]. Much of this outward journey was over decaying sea ice, which could break apart at any moment and sweep the men out to sea without hope of rescue [6, p 236]. They had no sled dogs, no cars, and no ponies. They began the British Antarctic Expedition with a car, but ironically, the car’s engine overheated and suffered ‘peculiarities’, and they were barely able to get any use from the machine [6, p 159].
![Figure 5: Shackleton’s map of the Nimrod expedition [1]](https://static.wixstatic.com/media/b00437_758482b230094886957fcf67e81f535b~mv2.jpg/v1/fill/w_980,h_960,al_c,q_85,usm_0.66_1.00_0.01,enc_avif,quality_auto/b00437_758482b230094886957fcf67e81f535b~mv2.jpg)
Ultimately, the team used sledges, which they hauled using raw man-power [6, p 236]. It was a grueling process, and behind the times; as Norwegian explorers were using sled dogs. The decision to man-haul was a distinctly British behavior. British saw dogs as pets, and were averse to the concept of using dogs as work animals or killing them for their meat when the situation called for it.
On January 16, 1909, the three men reached the pole within a radius of uncertainty based on the measurements made with their dip compass [6, p 244]. The team planted a flag, gave three cheers for the king, took a photo, and left. It was a momentous achievement, but similar to those trekkers who summit Mount Everest, the curling fingers of death were wrapping ever tighter around the explorers, and to linger would be to succumb.
After reaching the magnetic pole, they had fifteen days to trek 250 miles back to the Nimrod rendezvous point, or else be left behind on the frigid continent [6, p 245]. In the frantic race to get back to the ship, Mawson fell down a crevasse while David Edgeworth’s health began to fail [6, p 247, 272]. Fortunately, all of the men survived, and were picked up by the Nimrod, despite being several days late to arriving at the rendezvous point.
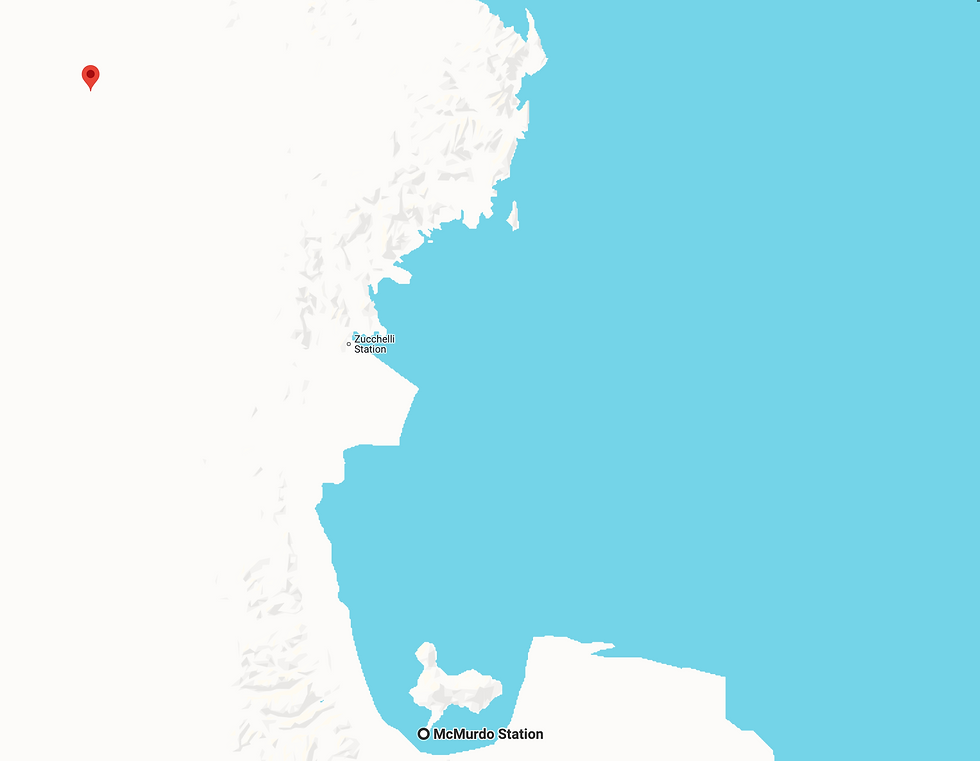
If you are curious, here is a link to a Google Map of the Northern party’s journey.
The magnetic south pole has moved dramatically since then, and is currently in the cold waters of the southern seas [2].
References
[1] British antarctic expedition, 1907-1909 nimrod, ernest shackleton. URL: https://www.coolantarctica.com/Antarctica%20fact%20file/History/Ernest%20Shackleton_Nimrod_expedition.php.
[2] Nov 2024. URL: https://www.ncei.noaa.gov/products/wandering-geomagnetic-poles.
[3] Jeannie Evers and Emdash Editing. Core. Education, Jun
[4] David J Griffiths. Introduction to electrodynamics.
Cambridge University Press, 2017.
[5] Libretexts. 5.1: Earth’s interior, Sep 2022. URL: https://geo.libretexts.org/Courses/Lumen_Learning/Book%3A_Earth_Science_(Lumen)/05%3A_Earths_Interior_Processes/5.01%3A_Earths_Interior#:~:text=Convection%20currents%20within%
Comments